Los Alamos: Beginning of an Era 1943-1945
Designing the Bomb
The world’s first man-made atomic explosion took place only 28 months after the arrival of the first scientific contingent at Los Alamos. Few greater tributes to human ingenuity have ever been written. The theoretical basis for nuclear weapons was already understood, in its outlines, when the Laboratory was established. Many of the engineering problems were foreseen in a general way, but much remained to be done. The following summary of weapon theory (all of it known in early 1943) will serve to suggest the enormous difficulty of the task that lay ahead.
The nucleus of an atom of uranium-235 contains 92 protons and 143 neutrons. When this nucleus absorbs an additional neutron, it becomes unstable and usually divides approximately in half. The two fragments become nuclei of two lighter elements, having a total mass somewhat less than the mass of the original uranium nucleus plus the additional neutron. Most of the mass difference between the original material and the products is converted into kinetic energy–rapid flight of the fragments.
The product nuclei emit several neutrons (in 1943 nobody knew exactly how many, on the average) for every U-235 nucleus split. Other U-235 nuclei may absorb these neutrons and undergo fission in turn, producing still more neutrons. Such a chain reaction will proceed very rapidly, as long as one neutron (at least) from each fission causes another fission.
Theoretically, the energy release from one kilogram (about 2.2 pounds) of U-235 would equal the energy release from the detonation of 17,000 tons of TNT.
Given these facts, the problem was to devise a bomb deriving its explosive energy from the fission of uranium-235 (or of plutonium-239, the only other fissionable material under consideration in 1943).
Neither U-235 nor Pu-239 was available in sufficient quantity to make a bomb. It was thought that these materials would become available within two years. The task of the Los Alamos staff was to design the bomb, and to devise methods of manufacturing it, in advance of the scheduled deliveries of the needed material.
A separate and remarkable story lies behind the fact that the scheduled deliveries were made on time. The story being told here will limit itself primarily to what happened at Los Alamos. The Los Alamos task was to discover means of making the desired explosive liberation of energy take place efficiently and at the right time. A veritable mountain of difficulties stood in the way.
No one knew how much fissionable material had to be put together to support an explosive chain reaction, but it was known that the reaction could not occur if the amount were insufficient. The burning of conventional explosives is a chain reaction of a different kind; a tiny quantity of TNT burns as readily as a larger amount. Fission chains cannot occur in the saline way, because the neutrons on which they depend must remain within the fuel until they encounter fissionable nuclei. If the surface area of the fuel mass is large compared to the volume (i.e., if the fuel mass is too small or too much flattened out), then the neutron escape area is too large compared to the neutron source volume, and too many neutrons will find their way out without causing fission.
By now it is possible to determine mathematically how much fuel is enough, but only when the shape, density, and purity of the fuel material are known. In 1943 additional unknowns stood in the way. The exact average number of neutrons emitted in fission had not yet been measured. Neither had the pertinent “cross sections.”
The term “cross section” is an extremely useful one in the study of nuclear reactions. It is a measure of the 1ikelihood that a certain reaction will occur, stated in terms of effective target area. Perhaps the following analogy will help to make this clear:
A popular carnival game is one in which the customer throws baseballs at ranks of woolly dolls. If we suppose that the solid portion of each doll presents a front surface of one square foot, and if we neglect the diameter of the baseball, then the effective area, (“cross section”) of each doll, for the reaction we might call “direct hit,” is one square foot. But if we investigate other possible reactions, we find the effective area of the same doll changing. Assume, for instance, that only one direct hit out of two causes the doll to fall down. For the “knock-down” reaction, then, the cross section of each doll is .5 square foot. If we throw ping pong balls, the “direct hit” cross section may remain one square foot, while the “knockdown” cross section becomes zero. Or, if the fringe on the doll is unusually stiff, so that a baseball passing through the fringe some times causes the doll to fall, then the doll’s cross section for baseball knockdown may rise toward two square feet. And the knockdown cross section will be different for baseballs of different speeds. Cross section is the effective target area for a specific reaction or event.
If we consider a free neutron, traveling through a sphere of pure U-235 metal, we need to know how likely it is to cause fission, instead of escaping from the sphere. We need to know, in other words, not how large the U-235 nucleus actually is, but how large a target it presents statistically, for the fission reaction, to a neutron of given velocity. Fission cross sections of U-235 for neutrons of a wide range of velocities (energies) needed to be known before a bomb could be designed. Also, since the uranium would not actually be pure U-235, it was necessary to know the cross sections of various impurities, especially U-238, for neutron absorption without fission.
Only on the basis of careful experiments and measurements could the needed cross sections be learned. Particle accelerators (atom smashers) were used in these experiments, because they could produce, indirectly, beams of neutrons with which to bombard samples of bomb material.
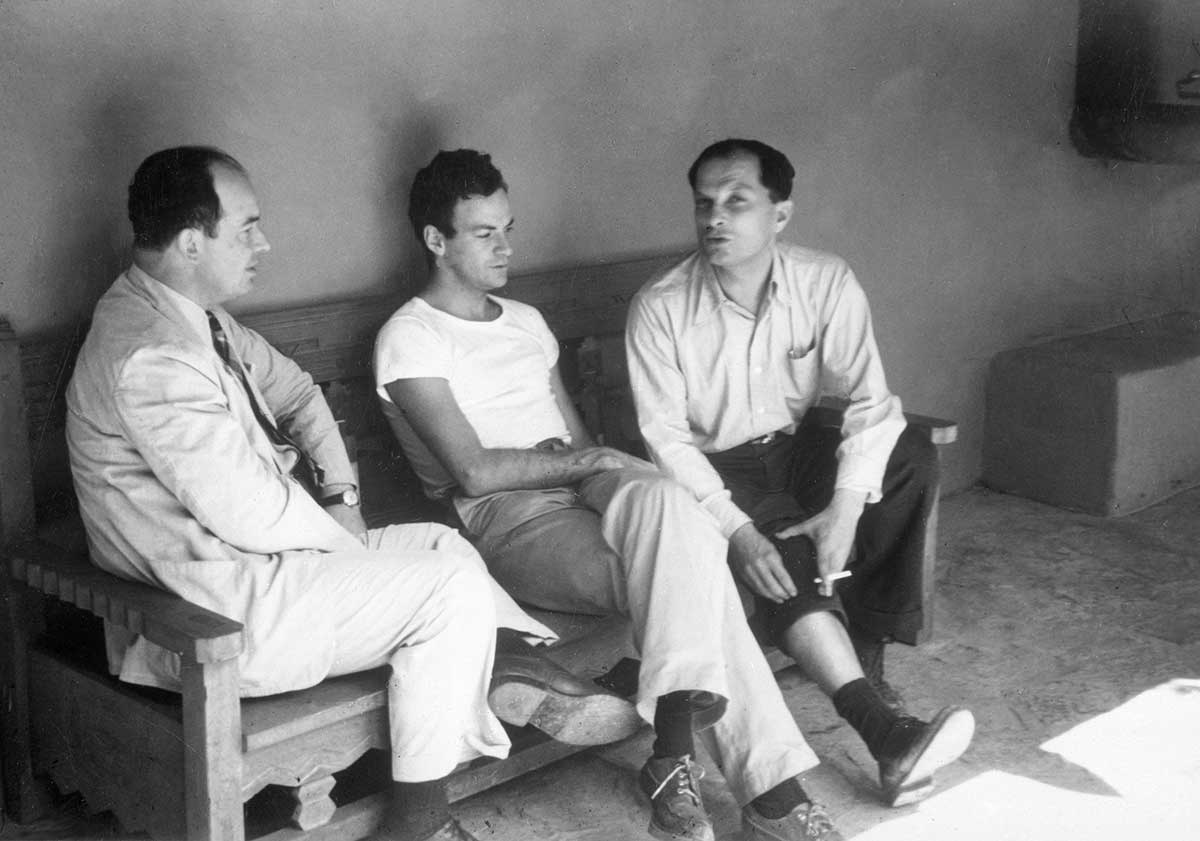
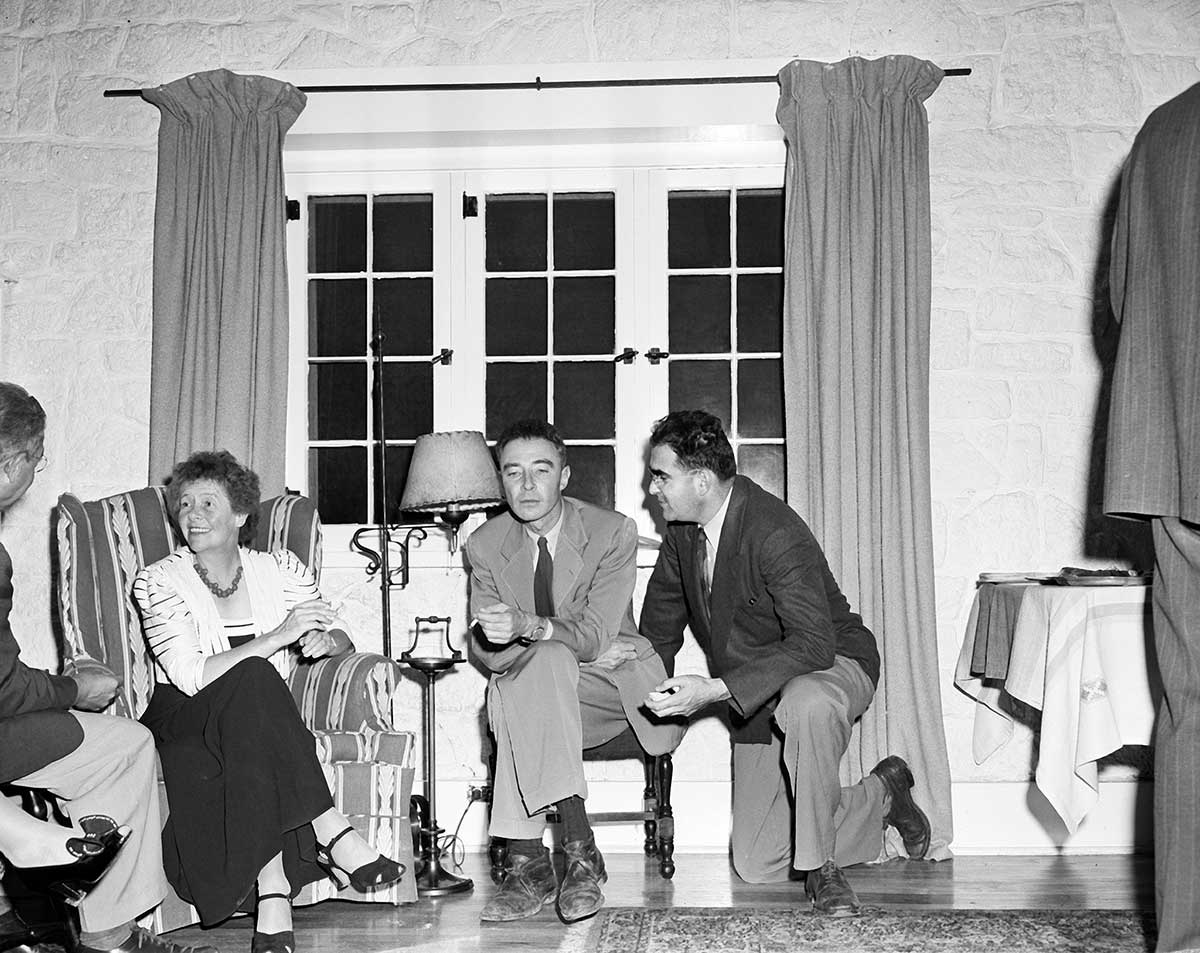
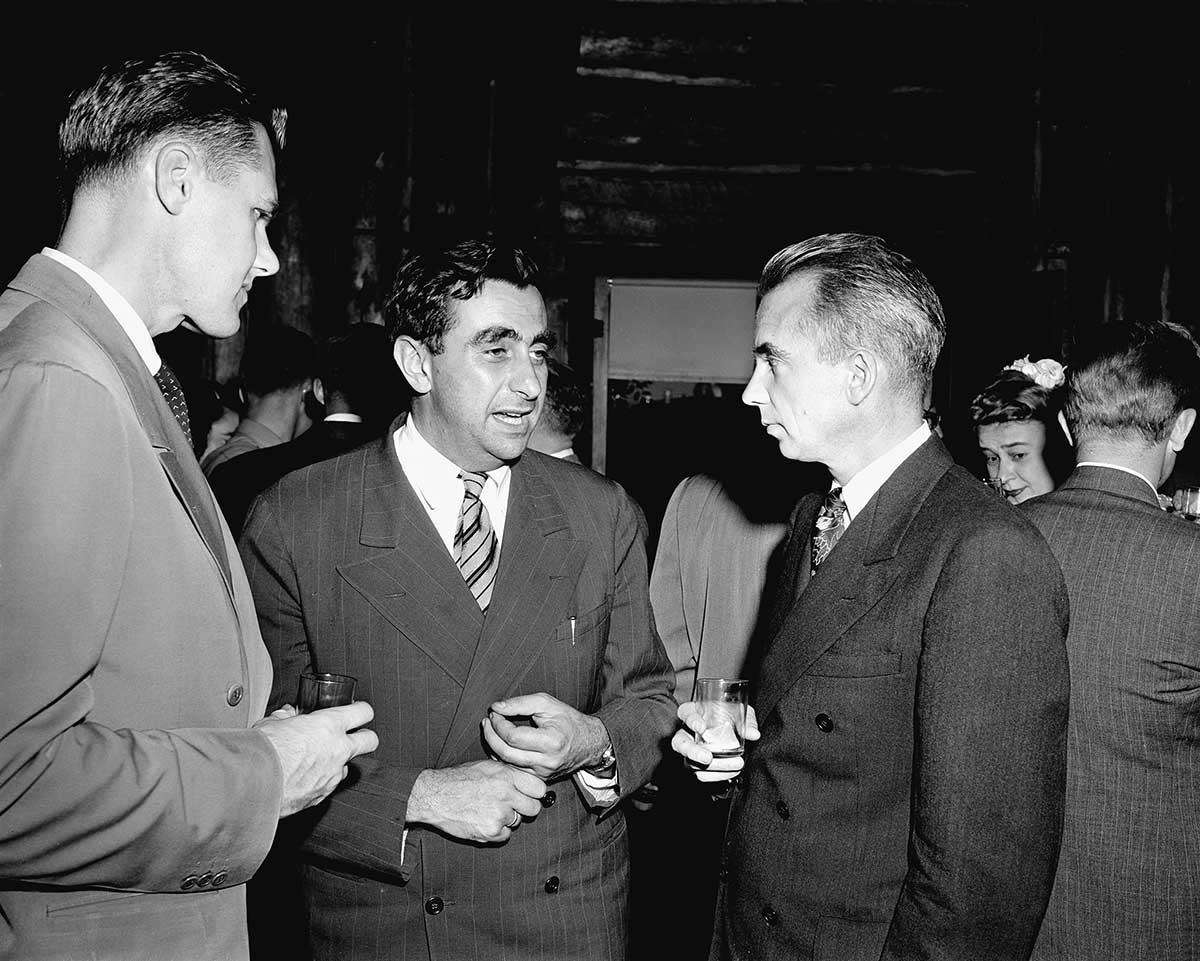
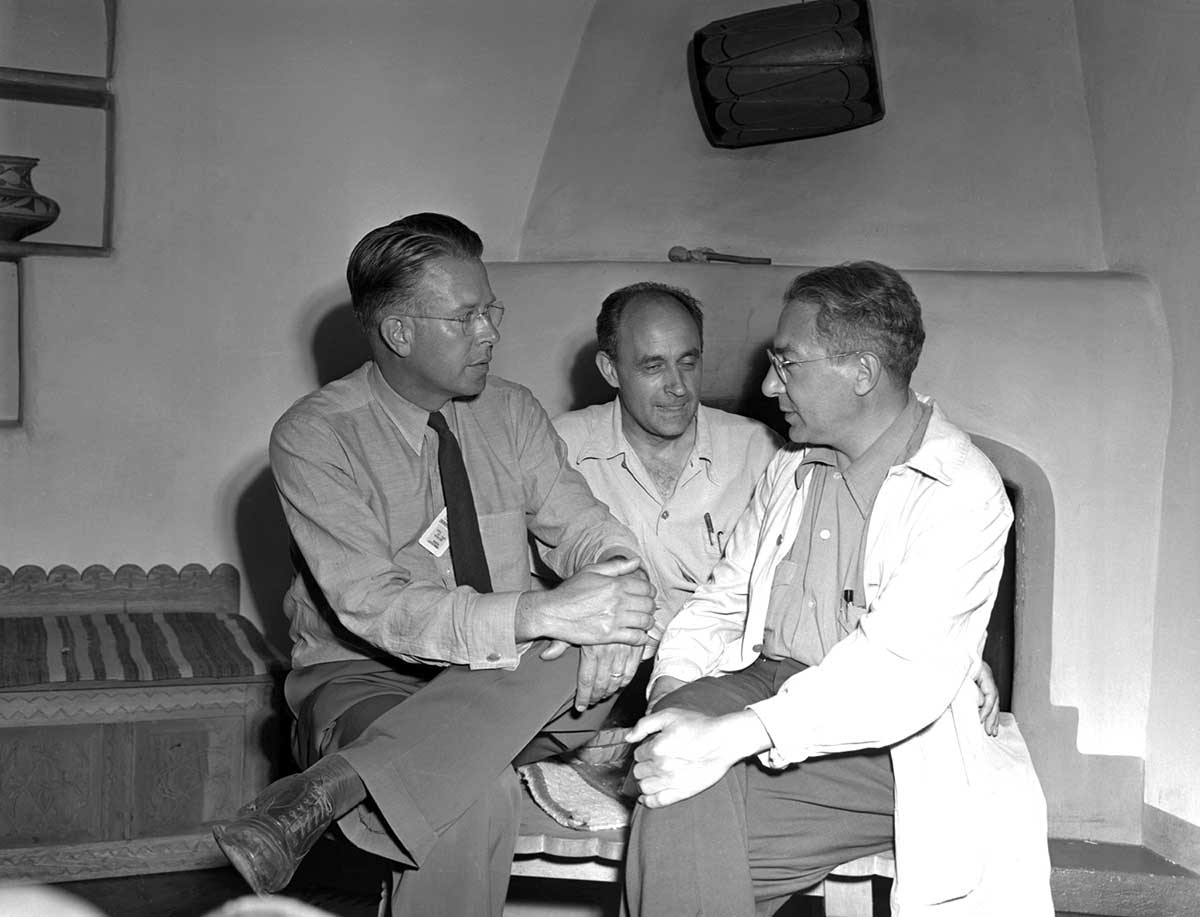
The mass of a sphere of fissionable material just sufficient to sustain a chain reaction is called the “critical mass.” By surrounding this material with an envelope of other material, to bounce escaping neutrons back into the active volume, it was possible to improve neutron economy and thus reduce the critical mass. Such a reflecting envelope is some times called a tamper.
The tamper in a weapon serves a second purpose. As the fissionable mass expands during the explosion, it quickly becomes less dense, at the same time, its surface area increases. These two effects act together to “quench” the chain reaction, since they facilitate neutron escape and reduce the likelihood that a given neutron will encounter a fissionable nucleus. A massive tamper slows the expansion and allows more energy to be liberated before the reaction is quenched.
Tamper materials, especially their cross sections for capturing neutrons and for scattering neutrons back into the fissionable material, needed intensive study.
It happens that the fission cross section of U-235 is greater for slow neutrons than for fast (presumably because the slow, neutron spends more time near the nucleus.) In spite of this fact, the reaction in a bomb must depend almost solely on fast neutrons. This is partly because the neutrons produced in fission arc naturally fast, partly because an air-delivered bomb must be as light as possible (therefore not permitting the inclusion of moderating material to slow the neutrons), and partly because a slow-neutron reaction system would not have time to liberate a large enough fraction of the potential energy before the bomb blew itself apart.
Therefore, is was necessary to establish facts relating to the efficiency 01 a tamped atomic explosion produced by fast neutrons. And these facts had to be established in advance of the delivery of fissionable material for the first bomb-in advance, of course, of any atomic explosion at all.
For the sake of explosion efficiency, it was inadvisable to depend on “background” neutrons (free neutrons unavoidably present in the bomb at all times) to start the reaction. The only way to be sure the reaction would start fast, and at exactly the right moment, was to arrange an internal neutron source that would deliver millions of neutrons in a single burst at the instant of complete assembly. Devices called “initiators” had to be developed to supply these neutrons.
As if such difficulties were not enough, a whole new supply of problems was introduced by the need to make the bomb go off at the right time and only at the right time.
A stick of dynamite is capable of exploding. When its cap or igniter sets it off, it explodes. A critical mass of fissionable material is not only capable of sustaining a chain reaction; it is incapable of not doing so. No percussion cap is necessary. Nobody lights a fuse. Once the critical conditions exist, the reaction begins. (This is because the one free neutron needed to trigger the reaction will always be supplied within a fraction of a second by neutrons from cosmic rays, spontaneous fission, or other sources.) Therefore, the detonation of a nuclear bomb occurs whenever its core is actually and fully assembled for the first time. The final assembly must occur only at the target. To say that this introduces a problem is putting it mildly.
Furthermore, the final assembly must be accomplished rapidly. As the core passes from its subcritical, or safe, configuration to its supercritical, or explosive, configuration, it must inevitably pass through configurations that are barely critical. Fast assembly is necessary because there must be no time for the reaction to occur and destroy the bomb before the optimum configuration is reached. Since no assembly method would be fast enough unless i t made use of high explosives, an intensive study of the potentialities of chemical explosives for this purpose had to be made.
In principle, two general methods of assembly appeared possible. One was the so-called “gun” method, in which one subcritical mass of fissionable material would be fired as a projectile at a target consisting of another subcritical mass of fissionable material. When projectile met target, the two together would constitute a supercritical mass. (The gun, with its explosive charge and its fissionable projectile, would have to be enclosed in the bomb casing, along with the target.) The other assembly method was “implosion,” in which a slightly subcritical mass of fissionable material would be surrounded by high explosives. When these explosives were detonated, they would compress the fissionable material, thereby increasing its density (decreasing the distances between target nuclei), thus rendering it supercritical.
The gun method appeared to be the easier to develop. It involved principles already well understood by ordnance experts, while the implosion method introduced entirely new principles of guiding explosive energy It was hoped that the gun method might work for- both uranium and plutonium bombs. It was a somewhat slower detonation system than implosion was, but its development would require fewer technological innovations.
In 1944 came the verification of a piece of bad news rumored a little earlier: The gun method was unsuitable for plutonium bombs. The reason was that plutonium produced in nuclear reactors (such as those at Oak Ridge and Hanford) contained a significant percentage of an isotope identified as Pu-240. Plutonium of this mass number had a strong tendency toward spontaneous fission, releasing neutrons. This produced an unusually high neutron background in plutonium containing the 240 isotope. Therefore, assembly of a plutonium bomb would have to be lightning-fast to prevent premature initiation of the chain reaction. Assembly by the gun method would be too slow; in a plutonium bomb, it would have to be implosion or nothing.
The simplest way to proceed might have been to build a few experimental bombs in the early nineteen-forties and try them out. Not the least of the Laboratory’s problems arose from the impossibility of doing this. By the time the precious shipments of fissionable material arrived at Los Alamos, a workable bomb design had to be ready. Various components and sub-assemblies could be tested by themselves, but no integral test of the weapon would be possible until long after the time when such testing might have served its purpose best.
As soon as the Laboratory had its first skeleton staff and a minimum of equipment (a cyclotron loaned by Harvard University, two electrostatic accelerators from the University of Wisconsin, a Cockcrow t-Walton accelerator from the University of Illinois, and much other borrowed equipment) the work began. In many ways it was a continuation of research already begun in a dozen laboratories, all over the country. But it had a focus for the first time. Los Alamos, and no other laboratory, would make the first bomb.
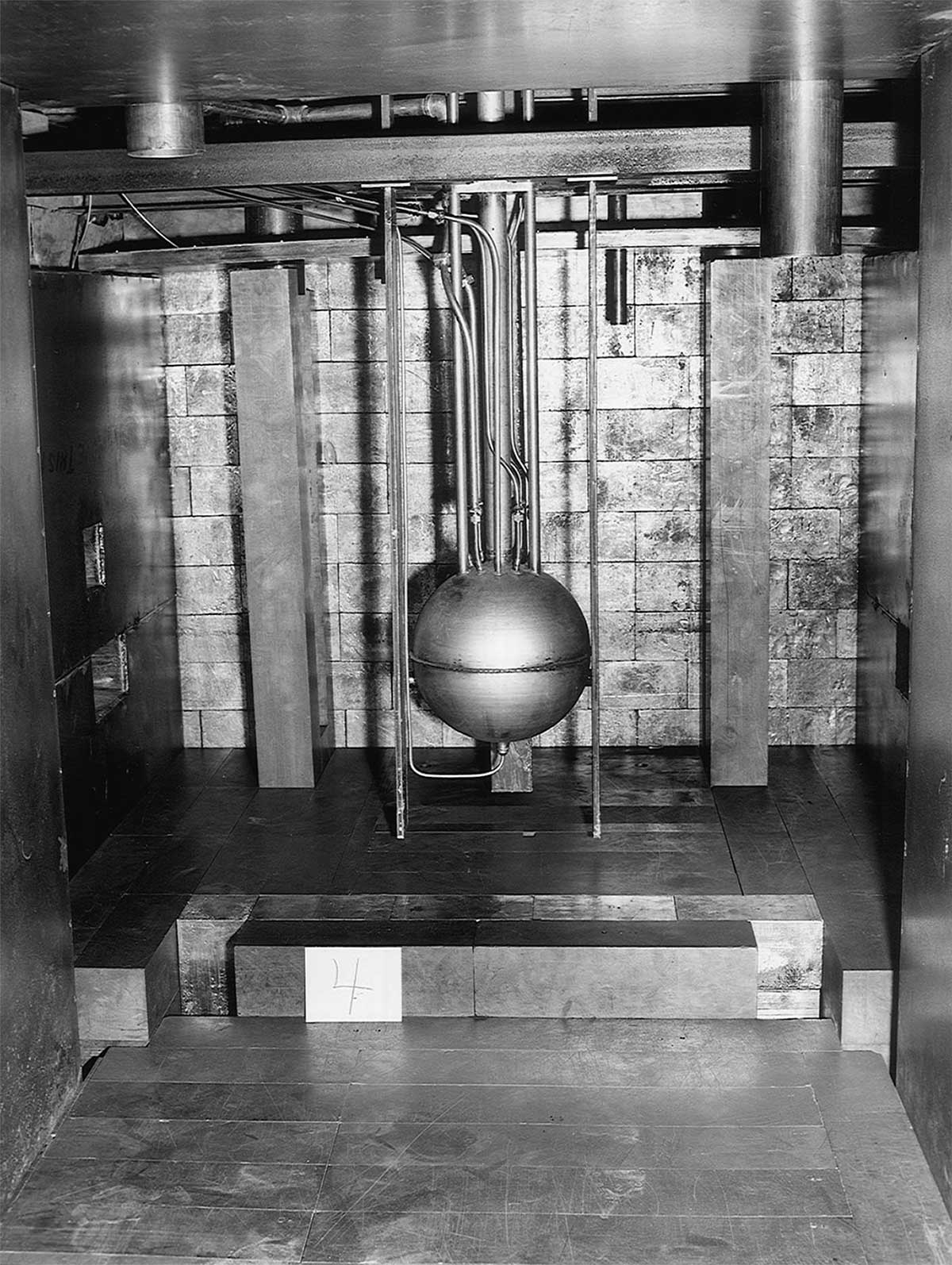
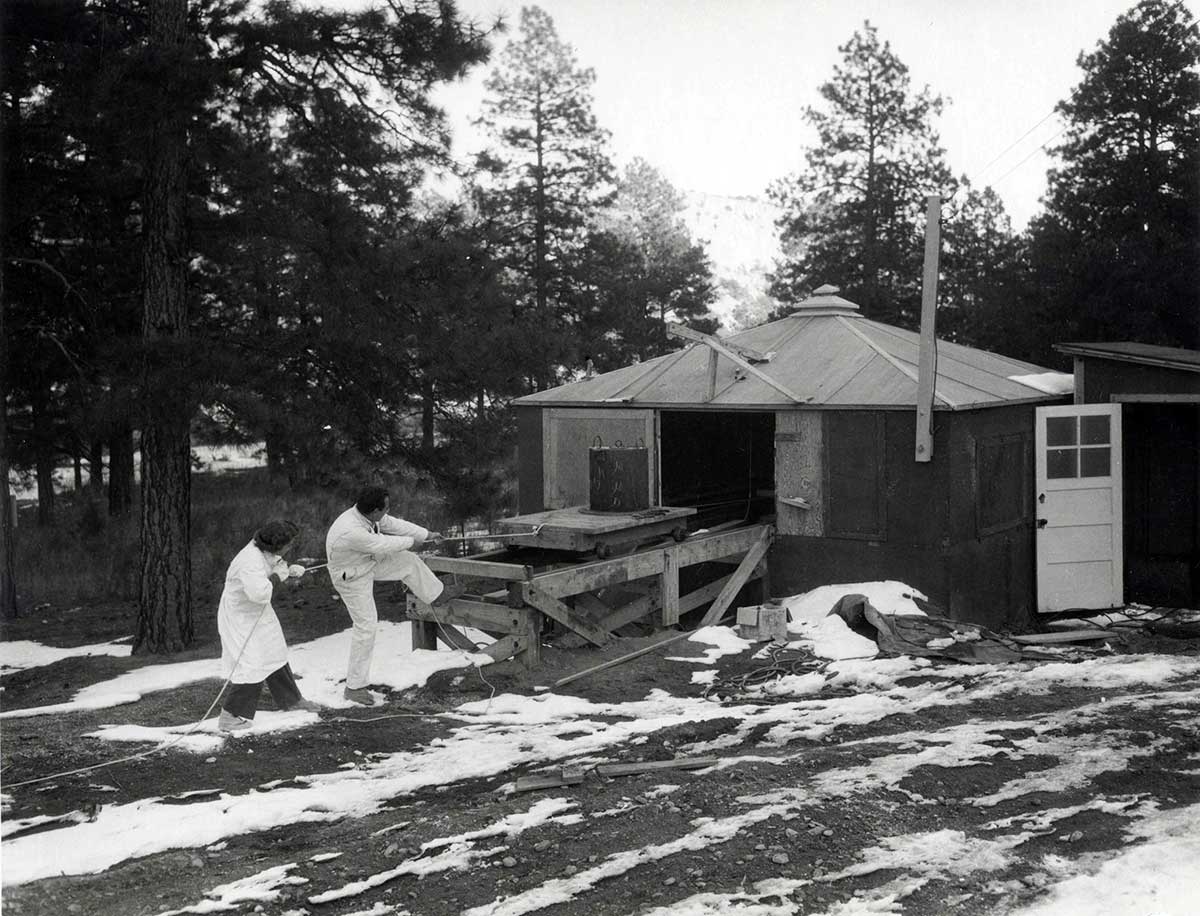
Research got under way on several fronts during the first half of 1943. Measurements of the “neutron number” (average number of neutrons emitted per fission) of plutonium-239 and uranium-235 were undertaken immediately, though the plutonium measurements had to be made on a sample scarcely visible to the naked eye.
Other research projects begun in the first months were these:
***Measurements of the fission spectrum (energy range) of neutrons from U-235.
***Measurements of fission cross sections Of U- 235 and Pu-239 for neutrons of high, low, and all intermediate energies.
***Measurements of the time (a fraction Of a millionth of a second) between fission and the emission of virtually all the fission neutrons.
***Measurements of cross sections of neutron capture and neutron scattering in various possible tamper materials.
***Development of experimental techniques (including ways of producing. and counting neutrons of specific energies, measuring fission in various materials, and measuring certain non-fission reactions induced by neutrons.)
***Radiochemical studies aimed toward the development of an initiator (the neutron source mentioned earlier) for the bomb.
***Research on uranium hydride, an early possibility for bomb fuel, later abandoned.
***Research on the chemistry and metallurgy of uranium and plutonium, and of possible tamper materials (including development of purification processes and analytical methods for measuring small amounts of impurities).
***Research on projectile and target materials for the gun program.
***Planning for construction of a deuterium liquefaction plant at Los Alamos, to supply liquid deuterium for experiments useful in the development of a thermonuclear bomb.
***An intensive ordnance program, studying the uses of high explosives for bomb assembly.
***A tremendous theoretical effort devoted to calculations of all kinds related to the physics and thermodynamics of the bomb.
At this early stage in the work of the Laboratory, it was believed that the development of the bomb would have two more-or-less distinct phases: (a) research in physics, chemistry, and metallurgy, then (b) technology in engineering ordnance design. The original directive set forth a plan for putting the Laboratory on a military basis in the second phase, commissioning the scientists in military rank, and so on. As it turned out, the commissioning plan was never put into effect.
In the fall of 1943, as a result of a conference between President Roosevelt and Prime Minister Churchill, it was decided to assign about two dozen British citizens to work at Los Alamos among them were some of the world’s most distinguished scientists.
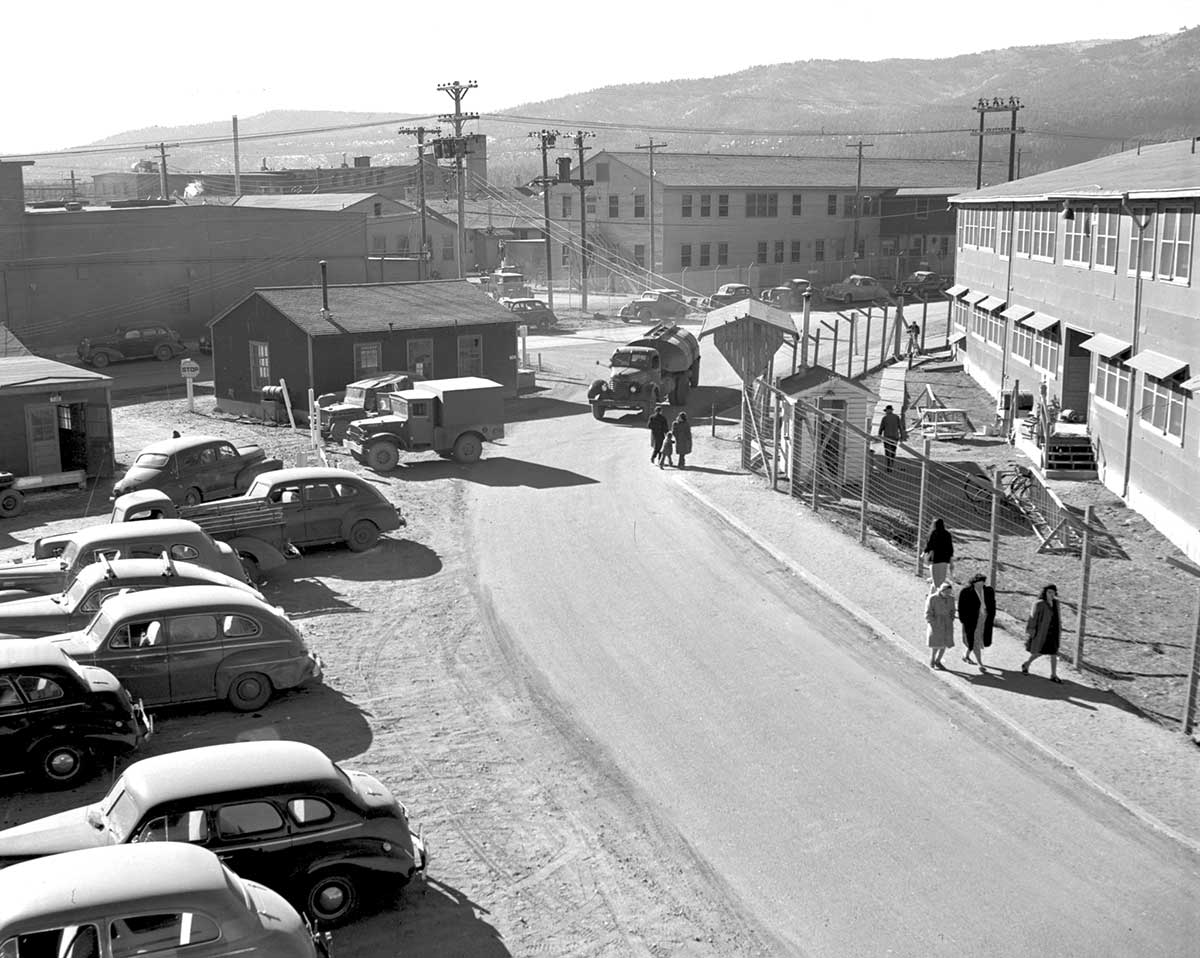
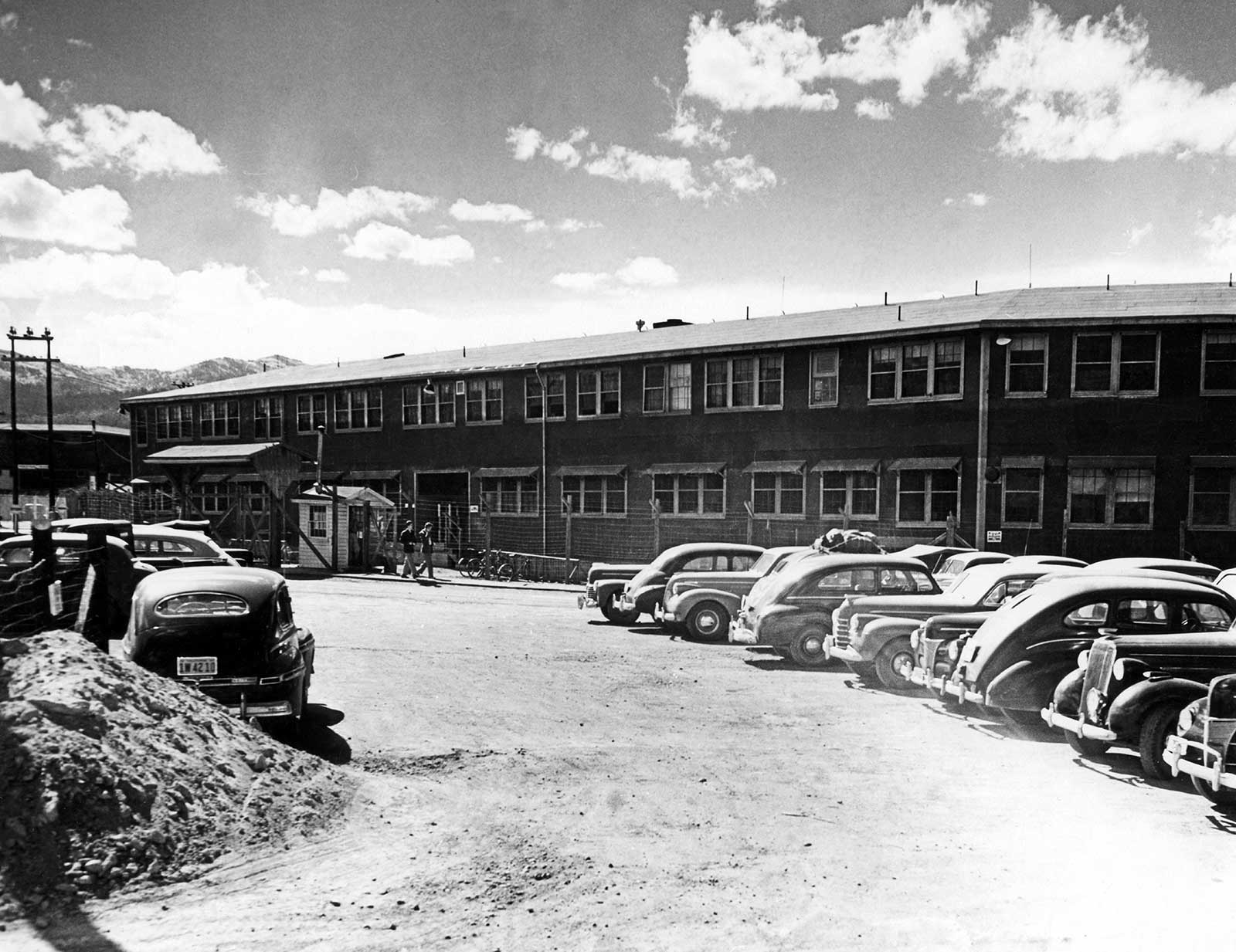
Laboratory personnel were all civilians until the fall of 1943, when a detachment of WACs and several technicians and scientists drafted into the Army’s Special Engineer Detachment (SED) joined the staff. By July, 1945, 50% of Laboratory personnel were military, mostly men of the SED. Total Laboratory personnel increased steadily from 250 in July, 1943, to 2,500 in July, 1945.
The most important single characteristic of Los Alamos Scientific Laboratory became apparent in the very first days. It has remained LASL’S most important single characteristic: The Laboratory is predominantly a scientific, not an engineering, institution. The fact that its first mission was the creation of a practical piece of hardware would seem to contradict such a statement, but the contradiction is only an apparent one. The nature of this specific piece of hardware was such that its creation called for a massive program of scientific research.
That it also called for a massive engineering program is equally true, but not central to the character of the institution as it was in 1943 or as it has been ever since. Every development program undertaken by the Laboratory has been of such an advanced kind that the technological effort was smaller, in terms of man hours, than the scientific one. It is impossible, of course, to separate the two kinds of effort in a clear-cut way, but anyone who has worked in the intellectual climate of Los Alamos knows that “Los Alamos Engineering Laboratory” or even “Los Alamos Research and Development Laboratory” would have been a misnomer-. “Scientific” is right, and the word was inserted in the earlier name, “Los Alamos Laboratory” in 1947.