Los Alamos: Beginning of an Era 1943-1945
Preparing the Materials
Preparation of the two fissionable core materials, meanwhile was being accomplished elsewhere against almost overwhelming odds.
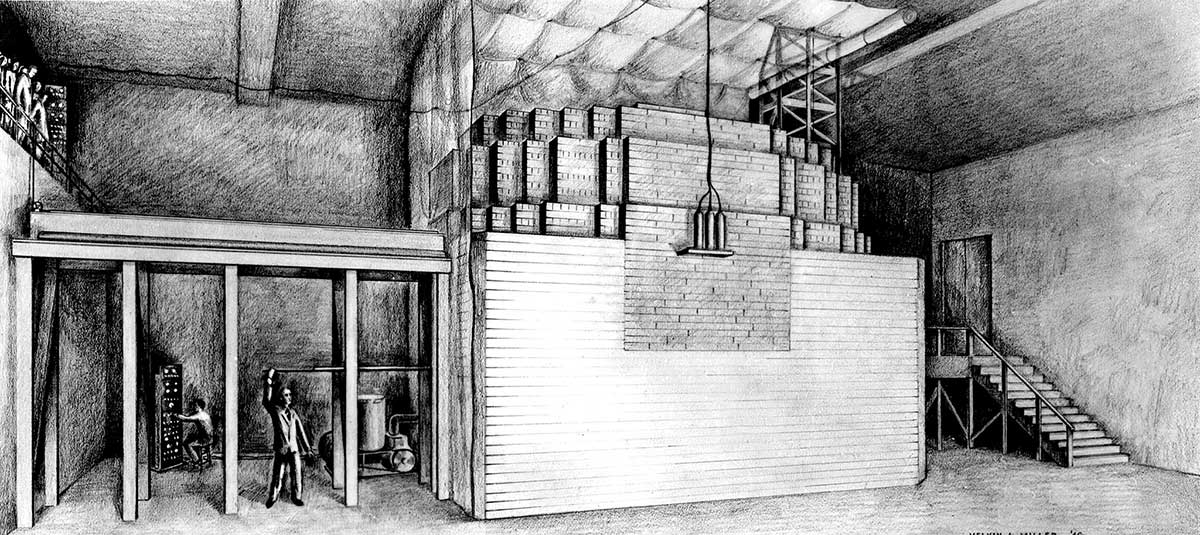
In the case of uranium, the difficulty arose from the fact that uranium 238 and uranium 235 are almost identical substances. Each of the two kinds of atoms has 92 nuclear protons and 92 orbital electrons. Since the chemical behavior of any atom is almost entirely governed by its orbital electrons, the two kinds of uranium could not be separated by chemical processes. Some other process—a purely physical one—was required.
U-235 has 143 neutrons in each nucleus. U-238 has 146. Somehow, those three extra neutrons had to be used to make the U-238 atoms go one way and the U-235 atoms go another. A great many methods were suggested. Half a dozen or so, including a centrifuge process like the one used to separate cream from milk, were given extensive trials, Almost every idea worked, but no idea worked very well. The difference between the two isotopes was too small.
Furthermore, all of the separation methods tried were expensive. If the isotope separation program had been an industrial enterprise, aimed at making a profit, the only sensible course would have been to close up shop.
But there was a war on. Nobody knew how much effort, if any, Germany might be devoting to nuclear weapon development (actually it was very little), but one thing was almost certain: If the Germans were first to develop a nuclear weapon, Hitler would win the war. This was no time to pinch pennies.
America’s decision, based partly on extremely good work by scientists in Britain, was to continue at any cost. Investigation of many ways of separating isotopes would go on, and a really vast effort would be made on the two most promising processes. One of these was gaseous diffusion separation and the other was electromagnetic separation.
Both processes are based on the difference in weight (more properly, in mass) between the two kinds of uranium nuclei.
The molecules in a gas are in constant motion. The warmer the gas, the faster its molecules move; but some move faster than others. On the average, heavier molecules are more sluggish than light ones. They move more slowly. Therefore, when a gas diffuses through a porous barrier, the lighter molecules get through a little more often (at first) than the heavier ones.
Perhaps unfortunately, uranium is not a gas. For the gaseous diffusion process, the uranium has to be combined with fluorine to produce an easily-vaporized compound called uranium hexafluoride. Uranium hexafluoride gas is extremely corrosive, tending to attack pumps, piping, barriers, and almost anything else it happens to touch.
But the gaseous diffusion method works. Passage through each barrier in a multi-stage separation plant increases (very slightly) the concentration of U-235 in some of the gas. By using thousands of stages, thousands of miles of piping, and hundreds of acres of barriers, it is possible to produce very highly enriched uranium hexafluoride. Uranium metal made from the enriched gas has a very low concentration of U-238.
A large part of the wartime project consisted of planning and building a separation plant to employ the principle just described. The plant was built in Oak Ridge, Tennessee, in the years from 1943 to 1945.
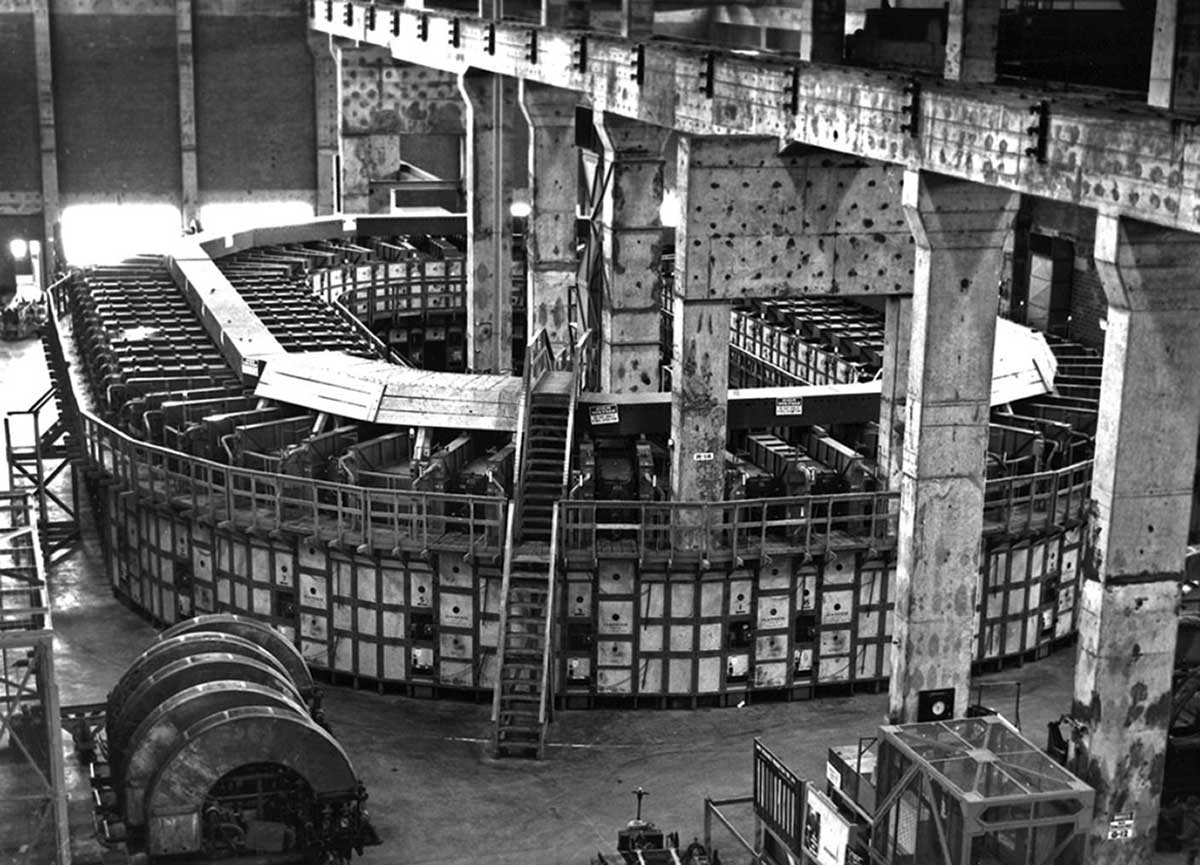
The electromagnetic separation process is quite different, but it also exploits the very slight mass differences created by the presence of those three extra neutrons in each U-238 nucleus.
Everything possessing mass has inertia. The more mass, the more inertia. It is perhaps usual to think of inertia as a reluctance to move, but inertia is a broader phenomenon than that. It is less a resistance to movement than a kind of resistance to change. If an object is stationary, inertia makes it reluctant to move; if it is moving, inertia makes it reluctant to stop or to change direction.
Since inertia is proportional to mass, the U-238 nucleus has a little more inertia than the U-235 nucleus. If both are traveling at the same speed, the heavier nucleus will have a slightly stronger resistance to any change in direction. Therefore, a given force tending to change the direction of motion will have a slightly greater effect on the lighter nuclei than on the heavier.
This principle is exploited in the electromagnetic separation of isotopes in the following way: First, usually by being the uranium atoms are “ionized,” deprived of one orbital electron each. This leaves the atoms positively charged, so that they can be accelerated electrically and acted on magnetically. When they have been accelerated-many millions of them at a time—they are formed into a beam, all traveling in the same direction. The beam of uranium ions is then passed through a magnetic field which has been arranged in such a way as to bend their trajectories. Under the influence of the magnetic field, the U-235 ions change direction more than the U-238 ions. The beam becomes two beams, each of which can be caught in a separate receptacle.
Though the development of the electromagnetic separation process encountered many difficulties, the method ultimately succeeded in producing important quantities of U-235. Electromagnetic separating machines called “calutrons,” developed by the University of California, were installed at Oak Ridge. They were used mainly to increase the enrichment of already-enriched products of the immense gaseous diffusion plant and of a smaller thermal diffusion plant, which used uranium hexafluoride in liquid form.
By late 1944, highly enriched uranium compounds were being produced at Oak Ridge in kilogram quantities.
Meanwhile, the program to produce the previously unknown element of atomic number 94 had made great strides.
Berkeley scientists produced minute quantities of plutonium in the winter of 1940-41, by bombardment of uranium with particles from an accelerator. The new element proved to be readily fissionable, as had been predicted.
However, production in quantities of military significance could not be carried out with particle accelerators. What was needed was a really plentiful source of’ free neutrons. The only sufficient source would be a nuclear fission reactor.
Fission reactors are devices in which a chain reaction is maintained under controlled conditions. No such device had been built when the Berkeley scientists produced their first plutonium. It would take two more years to achieve the first man-made fission chain reaction.
The leader in that achievement was the same Enrico Fermi who had first split the uranium nucleus. He had come to the United States and was working at the University of Chicago.
Fermi and his associates sought to demonstrate the possibility of a fission chain reaction in natural uranium—uranium containing less than one per cent U-235. Though a natural-uranium reaction would release energy at a rate unsuitable for an efficient nuclear explosion, the demonstration that such a reaction could be maintained would have great significance. Among other things, it could lead to the construction of reactors capable of producing large quantities of plutonium.
In Fermi’s experiment, lumps of natural uranium metal and of natural uranium oxide were placed in a “lattice” (a system of regular spacing) within a pile of graphite blocks.
The graphite was necessary to enable the pile to sustain a chain reaction. Here is why:
When a uranium nucleus undergoes fission, neutrons come out at high velocity. In a natural uranium system, these high-speed neutrons collide with uranium nuclei of both kinds. Some of the collisions cause fission, but many others do not. Most of the neutrons become involved in a series of “elastic” (glancing or bouncing) collisions with nuclei. Such collisions do not cause fission, and each such collision robs a neutron of some of its speed.
It happens that the velocity of a neutron has a large effect on what the neutron can do to a uranium nucleus. As the velocity goes down, the neutron loses its ability to cause fission in U-238, while acquiring even greater ability to cause fission in U-235. At what is called “thermal” velocity (when the neutron has lost all of the initial impulse it received from the fissioning nucleus) its ability to cause fission in U-235 is very high.
Unfortunately, there is a certain intermediate velocity at which a neutron is most likely to be captured by a U-238 nucleus, without causing fission. In a chain-reacting pile using natural uranium, it is therefore desirable to prevent collisions between medium-speed neutrons and U-238 nuclei. Otherwise, so many neutrons will be captured that the chain reaction will die out. (It is exactly such captures that result in the formation of plutonium, but Fermi was not yet trying for that; his pile would require a maxium number of free neutrons, just to keep the chain reaction alive.)
By using lumps of uranium separated by blocks of graphite, it is possible to avoid many of the neutron captures that would occur in a structure of pure uranium. Neutrons produced by fissions in one lump of fuel fly out of that 1ump and into the graphite before they have lost enough speed to be captured readily by U-238 nuclei. In the graphite, they lose much of their velocity, because of elastic collisions with carbon nuclei. By the time the neutrons reach the next lump of fuel, they are “thermal” (slow), and are not so likely to be captured by the U-238.
Fermi’s pile produced its first sustained chain reaction in December, 1942, exactly one week after the Under Secretary of War had directed that a site at Los Alamos, New Mexico, be acquired for a nuclear weapon laboratory. Fermi’s success demonstrated the possibility of the sustained chain reaction and gave great encouragement to those who planned to use larger piles as neutron sources for the production of plutonium.
Construction of one such pile began in Tennessee in 1943. By November of the same year, it was in operation. Within a few months after that, it had produced several grams of plutonium.
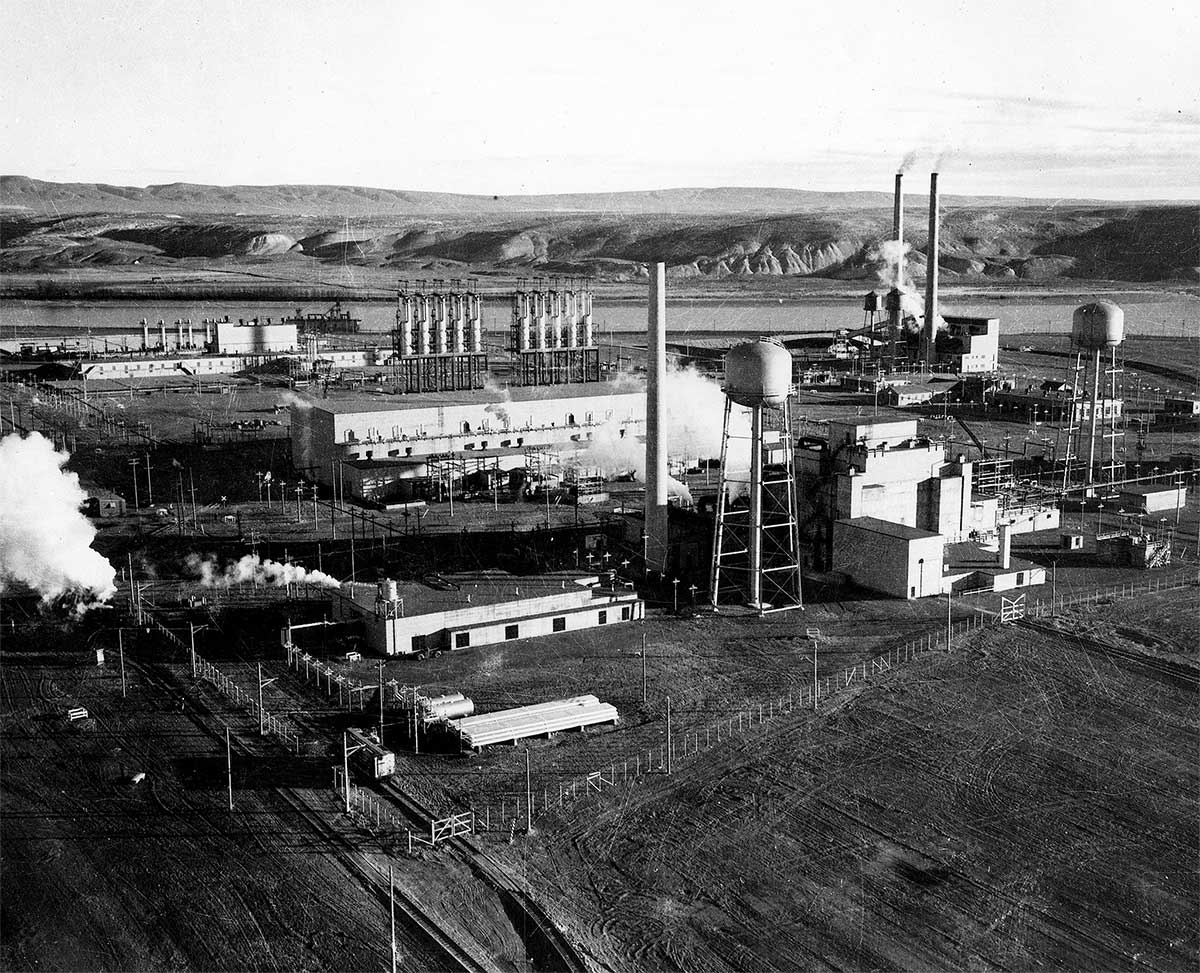
However, much larger plutonium production reactors would be necessary for the production of enough plutonium to be used in bomb cores. In June, 1943, construction of such reactors began at Hanford, Washington, where water from the Columbia River could be used as a reactor coolant. By September, 1944, the first Hanford pile was in operation. Plutonium nitrate from Hanford would soon join the flow of fissionable material that was already moving from the uranium and plutonium production facilities in Tennessee toward the Los Alamos Laboratory.
By September, 1944, the first kilogram of highly enriched uranium (63% U-235) had been received from the separation plant at Oak Ridge. By July, 1945, 50 kilograms had been received, and the enrichment had increased to 89%.
The first small quantities of plutonium (as nitrate, not as metal) arrived at the Laboratory in October, 1943. Gram amounts were delivered early in 1944, and soon after that still larger amounts began coming in, first from Oak Ridge and later from Hanford.
Both the uranium and the plutonium needed purification (an unprecedented job) before becoming suitable for weapons use. Means of purifying these elements were developed at Los Alamos and have been constantly improved.
The problem of preparing plutonium metal of high purity was started in the Laboratory in August, 1943 at a time when no plutonium was available for research. It gradually became available in amounts varying from micrograms to grams, but in the meantime, extensive preliminary investigations of possible methods of preparation had been made using other elements as stand-ins.
By the spring of 1944, the world’s first piece of plutonium metal prepared in any scale larger than a few micrograms was produced by the graphite centrifuge method which used centrifugal force to throw down molten metal into the tip of a cone during reduction. This was accomplished by placing the reaction mixture in a cone-shaped refractory liner sealed inside a steel “bomb.” The bomb was then placed in a graphite centrifuge which was heated rapidly to a high temperature while rotating. As the reduction took place the metal was thrown together in the tip of the liner, producing a good yield of coherent metal.
Meantime, however, research on the stationary bomb method indicated that gravitational force alone was adequate to separate the metal from plutonium fluoride, using calcium and iodine with appropriate heating conditions. The stationary bomb method, more suited to large scale production and much less complicated than the centrifuge method, was then adopted and is still used for routine production of pure plutonium metal. The centrifuge method, however, served its purpose at a time when it was most desperately needed.